The first time I laid eyes on a professional Sony OLED monitor I knew my professional life had changed. In a few years I’m sure we’ll take this technology for granted, but right now it looks AMAZING compared to any other monitoring system I use on a regular basis. For a slightly-technical-but-mostly-educational look at why, read on…
Until recently I knew nothing about LCD and OLED monitor technology, and being somewhat curious about such things I sat down with Sony product manager Gary Mandle to see if I could understand this seismic shift in digital monitoring.
This is how an OLED monitor works. Any questions?
First, though, a little history. If you already know the fundamentals of how CRT and LCD displays function you can skip to page 2. If not, it’s a good idea to read this page first so you can appreciate how different OLED displays are from anything that has come before.
The first successful broadcast television receivers were monochrome. They contained a vacuum-sealed glass tube through which an electron beam was fired at rows of phosphor dots placed on the inside front surface of the tube. Electron beams hitting the phosphors caused them to glow, and the brightness of the glow depended on the strength of the beam. Powerful magnets guided the beam on its path from the top left of the tube surface to the lower right, and this path was retraced 60 times per second. (For more detailed information on this technology check out the Wikipedia page on CRT tubes.)
Early phosphors didn’t glow for very long. Scanning the beam across the surface of the tube progressively, where every phosphor dot was hit in sequence, proved ineffective as the phosphors at the top of the screen dimmed noticeably before the beam had finished painting the full surface of the tube. This caused a massive roll bar where dimming phosphors chased newly lit ones. The solution-one that has haunted us to this day-was to illuminate the phosphor dots in alternating lines: the first pass of the beam illuminated rows 1, 3, 5, etc. and the second pass lit rows 2, 4 , 6, etc. Viewers didn’t notice that the interleaved odd lines dimmed as the even lines were lit, and the fact that the screen displayed 60 half resolution images per second instead of 30 full resolution images eliminated excess flicker. (50hz PAL TV sets appear to flicker to those of us who grow up in NTSC countries. Movie theaters reduce flicker by projecting each image twice as our brains see a lot of flicker at 24fps but not so much at 48fps.)
The same technology carried over into color television sets. Monochrome phosphor dots were replaced by a cluster of red, green and blue phosphor dots that fooled the brain into seeing a single color that was, in fact, a mixture of all three. For example, if the red dot was dim but the blue and green dots were lit the brain registered the combination as cyan. If the viewer got close enough to the screen they could see the individual phosphor dots, but at any distance beyond about 2′ they blurred into a single color. (The earliest tubes used a triangular cluster of RGB phosphor dots. Sony Triniton sets employed three closely-set vertical phosphor stripes.)
This system had some downsides. Early red phosphors weren’t bright enough to match the intensity of the blue and green phosphors so a small amount of green phosphor was mixed into the red to give it a bit more kick. Also, the response of the red phosphor was slower than the others so the addition of some green phosphor made it respond a bit faster. This phosphor mix resulted in reds having a slight orange cast. Most viewers didn’t notice.
Interlaced scanning reduced resolution considerably. Interlaced cameras capture images in two passes–odd rows first, then even rows–with a short but definite period of time between the two passes. Any object that moved significantly through the frame between those passes became blurred as the object appeared to be in one screen position for the odd field and another position for the even field. Even worse, noise in the system reduced resolution further as the odd field captured one pattern of noise and the even field captured another. When two fields with different noise patterns are combined the noise is amplified, reducing the resolution of the image.
Last but not least, black was created simply by turning the electron beams off. This meant that the blackest part of the image was no darker than the blank screen, which was not truly black. An unpowered TV tube surface appeared as dark gray, and while illuminating the phosphors around a dark area made that area appear darker by comparison there was no way to create a true, rich, deep black-especially ambient room light struck the front surface of the monitor and made it brighter. Instead of dark, rich blacks we had to settle for dark gray.
This most impacted color in shadows. As the picture tube’s native shade was dark gray, any color that approached “black” was contaminated by that gray. For example:
Bright colors overpower the gray base of the tube surface, but darker colors are impacted severely and are desaturated. We’ll see how OLED technology solves this problem shortly.
LCD displays tossed out the electron-beam-and-phosphor model and relied instead on some obscure properties of liquid crystal, which made it much more energy efficient but not as pretty as CRTs. The secret of LCD displays is that the liquid crystal is not responsible for creating the colors we see on our LCD television sets but is simply a light valve that allows light to pass through colored filters on the screen.
From back to front, here is a simplified description of how an LCD monitor is assembled:
(1) A light source (either a fluorescent tube, white phosphor LED or a set of red, green and blue LEDs) is placed at the rear of the display. This generates all the light that will pass through the display.
(2) In front of this backlight is a polarizing filter that polarizes the light.
(3) In front of that are two layers, one of circuitry (TFT) and another of liquid crystal. The circuitry layer divides the liquid crystal into a grid.
(4) In front of each grid “cell” is a filter that is red, green or blue. These replace the phosphor dots in a CRT monitor.
(5) In front of the RGB filter layer is another polarizing filter, but this filter is installed at a right angle to the first filter. Light can only pass through this polarizer if it is twisted into alignment by liquid crystal.
Liquid crystal acts as a “light valve” by twisting light between two oppositely-polarized filters.
Polarizing filters only pass light that is polarized in a specific direction. If two polarizers are stacked and one is rotated 90 degrees from the other, they’ll cancel each other out and pass very little light. That’s the key principal behind LCD displays: left on their own, the two polarizers prevent light from reaching the surface of the display. Unless something else happens, the display remains black-or as black as an LCD screen can get, which, as we will see on page 2, isn’t very.
Applying an electrical charge to a cell of liquid crystal grid causes that bit of crystal to “twist” the light that passes through it, polarizing it into alignment with the front polarizing filter. If a small charge is applied then the light is twisted only a little bit in the same direction as the front polarizing filter, so only a little light gets through. A full charge twists it completely, allowing all of the light to pass through. Liquid crystal is simply a light valve.
The construction of a typical LCD monitor. “TFT substrate” refers to the grid array that is employed to cause elements of the liquid crystal layer to “twist” light.
If that grid element is behind a red filter then the light that is passed through the front of the display appears as a red point. Repeat for every cluster of red, green and blue pixels on the screen.
While LCDs have proven very energy efficient there are few that are pleasing to the eye and fewer yet that can be used for critical image evaluation. The first problem is that the two polarizers never quite cancel each other out completely, so some light always passes through to the surface of the screen. This creates the same issue that we saw with CRTs, where no part of the screen is truly black, which reduces overall contrast as well as color saturation in shadow areas. LCD screens are slightly worse in this regard than CRTs.
Each kind of backlight has its own issues. Flourescent tubes and white phosphor LEDs generally have a broad-enough spectrum for reasonable color accuracy but not enough color energy in any one portion of the spectrum to reproduce strongly-saturated colors. RGB LED backlights tend to be very “peaky” as strongly-saturated dye LEDs emit very saturated light on very narrow wavelengths, as opposed to phosphor LEDs which tend to emit a broader spectrum of less pure light
The interplay between these light sources, the dye filters over the pixels, and the two polarizers can cause some very interesting color issues. There’s a popular line of “broadcast quality” LCD monitors that are, for example, not always the same color and rarely the proper color. I had the treat of viewing a white phosphor LCD panel next to a fluorescent-lit LCD panel on a recent shoot, and while the white phosphor LCD image looked pretty good the other monitor looked yellow-green by comparison. I don’t know if this is the interaction of the fluorescent backlight with the dye filters or the two polarizers interacting with each other, but either way it means that I don’t know what’s being recorded. This can be a negative drag on long-term employment prospects. (I’ve gotten into the habit of looking at all the monitors on the set-from the on-camera focus monitor to the director’s monitor, client’s monitor and playback operator’s monitor-and mentally averaging the results in order to understand what’s REALLY being recorded.)
LCD displays don’t “reset” the way CRTs do. A CRT phosphor will dim quickly if not re-illuminated, but LCD cells don’t reset automatically: they stay the same until they are overwritten. Once the liquid crystal behind a blue filter is partially depolarized, perhaps to display the color purple, the amount of light passing through that blue filter won’t change until the next frame, where different instructions may be issued. This can produce a “smear” effect. (The Sony BVM L231 monitor had a mode called “black frame insertion” where a black frame was inserted between each frame, shortening the amount of time that each video frame remained on screen. This increased the appearance of sharpness but also increased black levels.)
Last but not least, highlights have a habit of glowing. There are many additional layers in between the filters and your eye (I’ve not touched on all of them here) that scatter light as it passes through the display. Bright areas can bleed into dark areas, resulting in a halo that reduces the apparent sharpness of bright objects.
That’s enough history. Now for the good stuff…
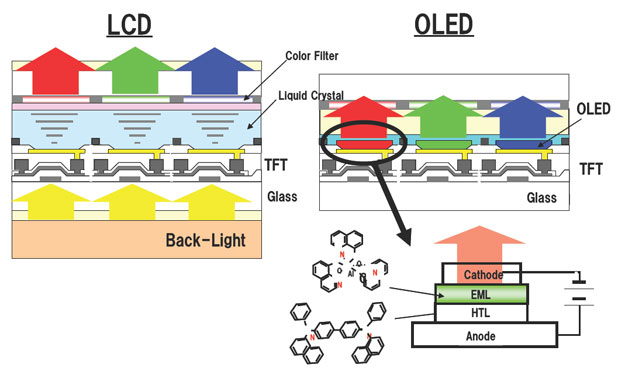
Light from an OLED display doesn’t have to punch its way through a liquid crystal layer or multiple polarizing filters. The color emitters are very close to the surface of the display to maximize their brightness.
The OLED display surface contains an array of red, green and blue organic material layers that glow brilliantly when an electrical current passes through them. These layers are arranged in clusters of tiny RGB dots (pixels) over a truly black surface that remains black at all times as there is nothing in the system (such as a backlight) to make it appear brighter. Emitted light passes through only a couple of thin layers of material before reaching the surface of the display so there is no opportunity for light to scatter and cause highlights to smear.
OLED displays are “emissive” systems: pure, bright color is emitted from points very near the surface of the display. LCD displays are “subtractive” systems: a light source at the rear of the unit is blocked by a system of polarizers, and light can only pass through to the front of the display if the liquid crystal allows it to.
This graph shows the deepest black that can be achieved with current CRT, LCD and OLED technologies. LCD blacks are not very black at all; CRT blacks are better but not perfect. OLED blacks are… well, pretty much perfect. (All monitors tested were manufactured by Sony. The CRT was a BVM A24E1WU, the LCD was a BVM L231, and the OLED was a BVM E250.)
Sony uses several interesting tricks to create colors that are so saturated that they can accurately represent large color spaces such as the digital cinema P3 color space. OLED materials create saturated colors, but Sony uses a combination of micro cavities and filters to make the colors purer still:
OLED microcavities.
The above illustration looks fairly complex but the concept is easy to understand. Light is released into a small cavity that is 1/4 the size of the wavelength that Sony wants the light to be. Over the top of the cavity is a funky quantum two-way mirror that allows 1/4 wavelength light to pass through it but reflects any light that has a wavelength that is longer or shorter. A fair amount of the light emitted is the correct wavelength and is quickly passed through the mirror but the rest is bounced around inside the cavity (“optical resonance”) until it becomes the proper wavelength. This dramatically increases the purity of the emitted color.
The final step is a colored filter that purifies the light just a bit more before launching itself toward your eyeballs.
During a recent demonstration at a Sony lab I was shown a digitally-created image in both the Rec 709 and P3 Cinema color spaces displayed on a PVM-grade OLED display. The Rec 709 image looked nearly black-and-white by comparison to the P3 image, which was simply dazzling. OLED displays are the first displays to display these large color spaces accurately.
Where OLED technology really shines, ironically, is in the shadows. CRT and LCD displays can never achieve true black, so as colors become less saturated they mix with gray–and gray contains equal amounts of red, green and blue, just as white does. Mixing a saturated color with white reduces its saturation considerably, and the same is true when mixing a saturated color with a darker white, or gray.
OLED screens are always black, and as black is defined as the absence of color there is no shift in saturation as a color gets darker. Color saturation is always the same.
This graphic, based on Sony tests, shows how color saturation diminishes as image brightness decreases and the colors blend with the gray of the LCD screen. An all-black LCD screen is surprisingly bright.
Sony OLED monitors don’t have the same issue: the surface of the monitor is black regardless of whether it is on or off. Colors remain saturated as they become darker. I can testify that this simulation is extremely accurate-or as accurate as can be shown on an LCD display.
Simulated image of the difference between an LCD image and a Sony OLED image. Based on my experience I can attest to the fact that this simulation is completely accurate.
Another advantage of a perfectly black screen is that shadow detail is beautifully rendered. By comparison a CRT or LCD monitor looks a bit like film that has been “flashed.”
Dark gray LCD “blacks” may reveal more detail in shadows by raising the black levels, but darker OLED blacks reveal more detail by increasing contrast and tonal separation for a much richer image.
Whereas LCD display contrast has typically been rated in ratios of 400:1 or 1000:1 (the ratio of difference in brightness between the brightest pixel and the darkest) Sony claims an OLED contrast range of 1,000,000:1. When I asked how the contrast could be so high I was told that the surface is SO black the contrast is almost infinite. If the number representing the dark end of the contrast scale is nearly zero then dividing that number into the brightest value results in a very, very high contrast ratio.
Contrast range by monitor type, based on Sony tests.
Simulated image showing increased contrast in an OLED monitor vs. traditional LCD technology. Yes, it looks that good.
OLED technology offers yet another distinct advantage: it is the only display technology that allows pixels to be turned completely off, quickly and on demand. CRT phosphors glow until they don’t anymore, and (little known fact) LCD pixels don’t change at all until they are specifically told to. OLED pixels can be turned on and off nearly instantaneously, in microseconds. This increases contrast in two ways:
(1) Pixels can reset to black nearly instantaneously, so black levels remain consistently as black as they can be.
(2) Contrast between frames is enhanced as the display system doesn’t add additional motion blur. LCD pixels don’t reset quickly, which creates the appearance of image smearing between frames. OLED’s response time is so fast that the only blur present is whatever was in the image in the first place. The display doesn’t add ANY.
Images appear sharper because the changes between frames happen instantaneously without interference from lagging LCD light valves. The only complaint so far with Sony OLED monitors is that they are so good that they bring back a phenomenon that we experienced last in the CRT days: flicker.
It’s easy to forget that a little over five years ago we learned how to tune out the flicker of a 24p (48i) image on a CRT. When Sony commenced development of OLED monitors they surveyed many in the industry and asked what they wanted in a monitor and were overwhelmingly told, “Make it look like a CRT!” They did-and now we’re complaining about flicker that, until recently, we accepted as normal. Sony is in the process of giving us what we really want by removing the flicker that many of us inadvertently asked for in the first place.
Simulated image, but still accurate.
One measure of a monitor’s response time is called the “gray to gray time,” or G2G. This is the amount of time required for a pixel to shift from middle gray to maximum white and back again.
This chart, based on Sony research, shows the dramatic improvement in G2G response time between OLED and LCD technology. Shorter bars are better.
This crispness is more important than ever as the world has largely transitioned to progressive image capture over interlaced scanning. Images are still stored and transmitted in two segments, but those two segments are split from a progressively-captured frame so no resolution is lost.
And while we’re on the subject of resolution I should point out once again the OLED dyes are mounted quite close to the surface of the screen, eliminating the halo effects that occur in LCD displays around very bright objects. Not only are images crisper and blacks darker, but highlights are very sharply delineated as well.
All this is to say that we are capturing temporally crisper images than at any other time in broadcast history, and with OLED technology we can now appreciate them fully.
Sony has broken their professional OLED lines into two branches. The BVM models are almost too good: they aren’t designed to be pretty (although they are) but are instead designed to reveal everything about an image, good and bad. Only the best monitors can be relied on consistently to reveal the tiniest flaws, and that’s what broadcast engineers require to ply their trade. The PVM line is what people like myself need for field work: a solid, reliable monitor with 10-bit processing (most LCD monitors are 8-bit), the ability to view multiple color spaces, and deep, rich blacks.
There may be a period during the LCD-to-OLED transition where the on-camera focus monitor will give us a better idea of what viewers at home will see because the OLED director’s monitor will look “too good.” I hope that transition period doesn’t last long. The one thing I look forward to more than anything else is setting up a large PVM-class OLED monitor in video village to dazzle clients and ensure a steady stream of repeat business. The second thing I look forward to is having a PVM OLED monitor mounted on the camera so I can actually see what I’m shooting.
Meanwhile, write your favorite television manufacturer and ask them to stop making 3D sets. For a truly transformative TV viewing experience, OLED technology can’t be beat. Stop by the Sony booth at NAB to see for yourself.
Thanks are due to Gary Mandle, Sony product manager for OLED monitors, for his generous assistance in the creation of this article. Any technical errors or gross simplifications are my fault, not his.
All images but one are courtesy of Sony, Inc. and used with permission.
Disclosure: I have worked as an unpaid consultant for Sony, but to date have not received any compensation or special deals on Sony equipment or services.
Art Adams is a DP who likes to work on the dark side. His website is at www.artadamsdp.com.
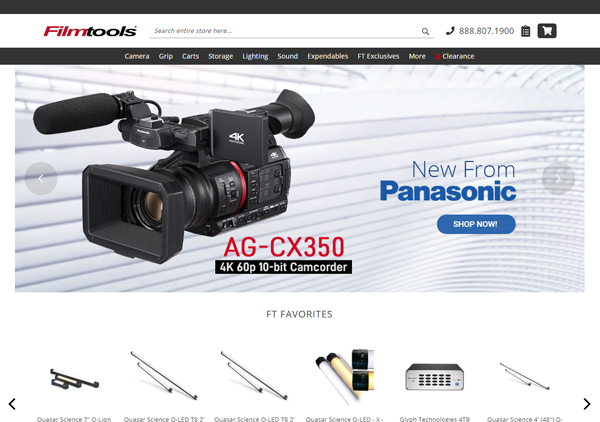
Filmtools
Filmmakers go-to destination for pre-production, production & post production equipment!
Shop Now